SCHYLER EDMUNDSON
How Mining Can Change the World: The Critical Role of Sustainable Resource Management in the Global Energy Transition
Author Schyler Edmundson
Written in Winter 2024 for SchylerEdmundson.ca
About: This reflective article explores the transformative potential of mining practices in the context of environmental sustainability. It highlights how the mining industry can adopt innovative technologies and methodologies to minimize its ecological footprint while contributing to the global transition toward a greener economy. By focusing on sustainable extraction, resource recovery, and responsible land use, the article argues that mining can play a crucial role in addressing climate change and supporting renewable energy initiatives.
​
Topics: Mining, Nuclear Energy, Sustainability, Natural Resources, Global Energy, Technology, ESG Compliance
​
Abstract: This paper explores the vital role of critical minerals in the global shift toward renewable energy, highlighting the complex interplay between mining’s environmental impacts and its contribution to sustainable energy solutions. As demand for minerals like copper, lithium, and uranium surges to meet renewable energy needs, the environmental footprint of mining processes poses significant challenges. Mining contributes directly to greenhouse gas emissions, water scarcity, and deforestation, yet it also provides essential resources for technologies like electric vehicles, wind turbines, and nuclear power. Chapter 1 addresses the global supply chain structure, illustrating the critical need for sustainable mining practices to minimize greenhouse gas emissions in energy-intensive processes, transportation, and waste management. Chapter 2 examines the potential of uranium mining for nuclear energy, particularly through small modular reactors, to complement intermittent renewables, offering insights into regulatory, safety, and geopolitical considerations. Chapter 3 evaluates the economic advantages of ESG compliance within the mining sector, showing how sustainable practices not only enhance financial performance but also position mining companies as leaders in the clean energy transition. This paper advocates for a balanced approach where innovative technologies, corporate responsibility, and international cooperation collectively transform mining into a sustainable cornerstone of global decarbonization efforts.
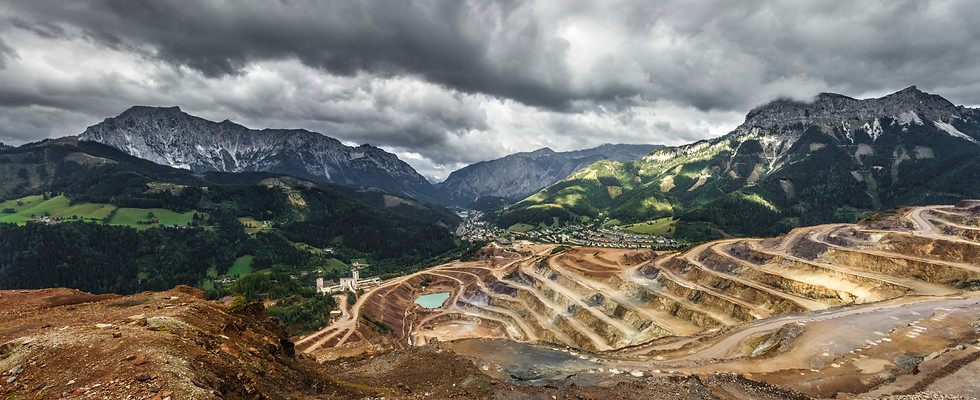
​Chapter 1: The Core Root of the Problem
​
Global Supply Chain Structure and Critical Minerals
Critical minerals play an essential role in the global transition to renewable energy. As the world shifts toward electric vehicles, solar power, wind energy, molten storage, and nuclear energy the demand for minerals like copper, lithium, and uranium is expected to skyrocket. According to the International Energy Agency (IEA), the supply of key minerals must increase by up to 500% by 2050 to meet the global demand for clean energy technologies (IEA, 2021). The World Bank predicts that over three billion tons of minerals and metals will be required to deploy wind, solar, and geothermal power, as well as energy storage (World Bank, 2020). Mining is thus integral to the climate solution, serving as the backbone for manufacturing the tools required to decarbonize the energy grid.
​
For example, copper is critical for wind turbines and electric vehicle (EV) charging infrastructure, while lithium and cobalt are essential for battery technologies (U.S. Geological Survey, 2022). Uranium, a key mineral for nuclear power, is increasingly being recognized for its role in providing baseload, low-carbon energy, which complements intermittent renewables such as wind and solar (IEA, 2021). Despite these contributions, mining also has significant environmental impacts, including GHG emissions, water use, and ecosystem disruption, necessitating a shift toward more sustainable practices (Ali et al., 2017).
​
Mining Process Impact on Climate Change
Mining operations are responsible for approximately 4 to 7% of global GHG emissions, with the majority of emissions stemming from energy-intensive activities such as ore extraction, crushing, and processing (World Bank, 2020). In copper mining, emissions can reach 4.9 tons of COâ‚‚ per metric ton of ore, largely due to the high energy demands of crushing and refining (Norgate & Haque, 2010). In regions like Latin America and Africa, which dominate global production of minerals like copper and lithium, mining operations also contribute to water scarcity and pollution through tailings and waste management (UNEP, 2020). The adoption of evolutionary and revolutionary technologies such as electric mining equipment could result in a dramatic reduction in mining’s carbon footprint. For instance, if a copper mine producing 5% of the global supply adopts low-carbon technologies by 2040, it could reduce its emissions by up to 2 million tons of COâ‚‚ annually, which is a significant step toward global decarbonization goals (IEA, 2021).
However, mining’s impact on climate change must also be considered in light of its essential role in providing the critical materials required for the global energy transition. Without mining, it would be impossible to produce the solar panels, wind turbines, electric vehicles, nuclear energy, and battery storage systems necessary to decarbonize the global economy. The key, therefore, is not to halt mining but to transform it into a sustainable industry that can continue to meet the growing demand for minerals while minimizing its environmental footprint. Here, I provide a comprehensive summary that includes both the current impact of mining on each greenhouse gas area and potential strategies to mitigate these emissions. For each production category, global statistics on greenhouse gas (GHG) emissions are included, along with examples of direct and indirect impacts from the mining industry, to suggest feasible solutions mining operations could adopt at each stage of problem emissions areas to address climate change.
​
1. Energy (Electricity and Heat Production)
The energy sector contributes about 25% of global GHG emissions, largely due to reliance on fossil fuels for power generation, which affects the mining industry significantly, as mining activities are exceptionally energy-intensive. Directly, mining relies heavily on electricity for tasks like crushing and grinding ores, and smelting operations for metals such as aluminum and steel, which emit vast quantities of COâ‚‚ when powered by coal-fired plants. Indirectly, remote mining locations often rely on diesel generators or even build dedicated fossil-fuel power plants, which adds to the emissions intensity of the extracted materials (IEA, 2023). For example, in Australia, where coal power remains prominent, aluminum smelting emits roughly 12 metric tons of COâ‚‚ per metric ton of aluminum produced. To address these emissions, mining operations could transition to renewable power sources, like solar and wind, for both grid-connected and off-grid sites. Studies suggest that renewable energy can reduce mining emissions by up to 40% by replacing diesel generation with clean power (Wood Mackenzie, 2021).
​
2. Transportation
Transportation is responsible for approximately 14% of global GHG emissions, with mining contributing through the movement of heavy equipment and materials across often remote areas (EPA, 2023). Directly, mining operations require massive vehicles and machinery, typically powered by diesel, to haul raw materials on-site and to transport extracted materials to processing facilities, which results in substantial emissions. Indirectly, the demand for minerals essential for electric vehicles (EVs) like lithium and cobalt increases transport emissions when these materials are shipped globally. For example, a single mining haul truck can emit over 3,000 tons of COâ‚‚ annually (McKinsey, 2022). Solutions such as electrifying mining trucks and adopting fuel-efficient rail and ship transport can significantly reduce emissions. Electrified haul trucks have shown potential for reducing mine-related transport emissions by up to 50% (McKinsey, 2022), and incorporating carbon-neutral shipping practices can further cut the sector’s footprint.
​
3. Industry (Manufacturing and Construction)
Globally, the industrial sector, which includes manufacturing and construction, contributes nearly 20% of GHG emissions, much of which can be linked to the mining and processing of metals (IPCC, 2021). Directly, smelting processes for iron, steel, and copper require intense heat, often provided by coal, which results in high emissions. Indirectly, mining supplies raw materials for construction industries, from concrete to copper wiring, further expanding emissions across these industries. For example, steel production alone releases over 1.8 metric tons of COâ‚‚ per metric ton of steel (World Steel Association, 2021). The adoption of hydrogen-based smelting, which can decrease emissions by up to 90%, or renewable electric arc furnaces could substantially mitigate these impacts (IEA, 2022). Additionally, carbon capture technology implemented at industrial sites has the potential to capture and store up to 95% of COâ‚‚ emissions generated (IEA, 2021).
​
4. Agriculture and Livestock
Agriculture accounts for roughly 18% of global emissions, but mining has indirect effects on this sector, particularly in terms of land degradation and deforestation, which displaces agricultural land and impacts soil carbon sequestration (FAO, 2022). Direct impacts occur when mining leads to land pollution and degradation, particularly through waste dumps or runoff, making soil less suitable for farming. Indirectly, mining expansion often results in deforestation, pushing agricultural activities further into untouched land, exacerbating emissions. In the Amazon, for example, mining for gold and other minerals has displaced vast amounts of forest, reducing its ability to act as a carbon sink (WWF, 2021). Solutions like reforesting mined lands or adopting regenerative mining practices that stabilize soil and restore ecosystems could reduce indirect impacts on agriculture. Regenerative practices are projected to increase carbon sequestration by approximately 10% when applied to degraded lands (FAO, 2022).
​
5. Deforestation and Land Use Change
Land use changes, including deforestation, are responsible for about 10% of global GHG emissions, and mining plays a direct role in this sector by clearing large forested areas for operations (IPCC, 2021). Mining often results in deforestation, releasing stored carbon and preventing future sequestration by healthy ecosystems. Indirectly, disturbed lands can suffer soil erosion and biodiversity loss, reducing the capacity of the landscape to recover and store carbon. In Indonesia, for instance, nickel mining has led to significant deforestation in rainforest areas, which are critical carbon sinks (Greenpeace, 2021). Reclamation practices, such as reforestation of mined lands, could mitigate this impact. It is estimated that reforesting abandoned mines could potentially absorb up to 0.5 metric tons of COâ‚‚ per hectare annually (UNEP, 2022). Transitioning to more eco-friendly techniques, such as underground mining, could also minimize the land cleared for open-pit mines.
​
6. Waste Management
The waste sector accounts for around 3% of global emissions, with mining waste (tailings and slag) contributing indirectly to these emissions (EPA, 2023). Directly, methane emissions can arise from mining waste, particularly when organic matter decomposes anaerobically in waste ponds. Indirectly, improperly managed mining waste affects nearby water and land ecosystems, which can influence biodiversity and carbon cycling. Coal mining tailing ponds, for instance, have shown significant methane releases due to trapped organic matter, adding to the GHG footprint (World Bank, 2021). Solutions such as using anaerobic digestion to manage organic waste and reusing slag and tailings in construction materials can reduce waste-related emissions. Repurposing tailings as construction materials, for instance, can cut waste emissions by up to 25% (World Bank, 2021).
​
7. Commercial and Residential Buildings
Commercial and residential buildings account for 6% of global GHG emissions, largely due to energy use for lighting, heating, and cooling, with mining contributing through its role in material supply (IEA, 2023). Directly, mining supplies essential materials like copper for wiring and steel for structures. Indirectly, however, emissions are amplified as extracted materials are processed and transported to construction sites. For instance, copper mining and refining produce around 3 metric tons of COâ‚‚ per metric ton of metal (Copper Alliance, 2021). Green building certifications, such as LEED, that prioritize lower-carbon materials could reduce this sector’s reliance on mining-intensive products, potentially lowering total emissions by 10-15% (USGBC, 2021). Additionally, using recycled metals for construction can reduce emissions by up to 60% per ton of metal (EPA, 2023).
​
Chapter 2: The Role Of Uranium Mining
Nuclear energy, despite its controversial nature and environmental risks associated with uranium mining, is emerging as a crucial component of the low-carbon energy mix required to mitigate climate change. Accounting for nearly 10% of the world's electricity generation, nuclear power provides a reliable baseload energy source, essential for balancing the intermittency of renewables like wind and solar (IEA, 2023). As the global community aims to meet ambitious climate targets, such as net-zero emissions by 2050, nuclear energy’s ability to produce power with nearly zero GHG emissions during operation positions it as one of the cleanest large-scale power sources currently available. This capacity for clean power generation is especially vital for energy-intensive sectors, such as mining, where nuclear energy could offer a stable alternative to fossil-fuel-based power.
​
A promising development in nuclear technology is the emergence of small modular reactors (SMRs), designed to offer the same power output as traditional reactors but with enhanced flexibility, safety, and scalability. These reactors are in various stages of development globally, with approximately 70 SMR designs under development as of 2023, and some already operational, including those in Russia and Canada (World Nuclear Association, 2023). SMRs can be deployed in remote regions, making nuclear energy accessible to areas lacking substantial energy infrastructure, such as mining regions in Africa and Latin America. By providing clean, consistent power in these locations, SMRs can reduce the carbon footprint of resource extraction while supporting regional energy independence. In addition to being quicker to build and requiring a smaller physical footprint, SMRs offer a scalable solution that can be gradually expanded based on demand, making nuclear power more feasible for reaching 2030 and 2050 climate goals.
​
However, the deployment of nuclear energy, particularly SMRs, requires stringent safety protocols and regulatory oversight to mitigate risks associated with nuclear waste, radiation, and potential accidents. The International Atomic Energy Agency (IAEA) has underscored the need for robust global nuclear safety standards, while various countries are refining regulations to balance innovation with public safety. For instance, Canada’s nuclear regulatory framework for SMRs emphasizes a stepwise licensing process, ensuring that each phase of development meets rigorous safety standards. As nuclear technology becomes increasingly decentralized with SMRs, consistent global safety standards and transparent regulatory practices will be essential to maintaining public trust and environmental protection.
​
The geopolitical implications of nuclear energy expansion are significant, especially as the demand for uranium — the key fuel for nuclear reactors — intensifies. With uranium mining concentrated in a few countries, including Kazakhstan, Canada, and Australia, there is potential for geopolitical tension over resource access and control. Uranium-rich regions must navigate complex environmental, social, and political challenges to ensure sustainable and ethical mining practices. Furthermore, an increase in nuclear reactors worldwide raises concerns around nuclear proliferation, as the spread of nuclear technology can be seen as both an energy solution and a potential security risk. International cooperation on uranium resource management and non-proliferation agreements will be critical to navigating the geopolitical landscape as nuclear energy gains momentum in the global energy transition.
​
In conclusion, while nuclear energy presents clear climate benefits, its expansion requires advancements in safety, regulatory frameworks, and international cooperation. The technology, particularly through innovations like SMRs, offers a viable path to decarbonizing energy-intensive industries and powering remote regions. With proper oversight and commitment to safety, nuclear energy has the potential to play a transformative role in the fight against climate change, supporting a resilient and diversified low-carbon energy mix.
​​
Chapter 3: Profitability of Corporate ESG Compliance
As the environmental and social impacts of mining come under greater scrutiny, the mining sector faces increasing pressure to adopt sustainable practices. This pressure is not only regulatory but also financial, as investors, governments, and consumers seek accountability in industries that significantly impact the planet. Embracing Environmental, Social, and Governance (ESG) compliance offers mining companies a pathway to improved financial performance, corporate reputation, and operational efficiency. For mine owners, transitioning to ESG-compliant practices not only supports global climate goals but also aligns with long-term business profitability and resilience.
​
ESG Financing Options and Corporate Compliance
One of the most immediate financial incentives for ESG compliance is access to new forms of financing. ESG-driven financing, such as green bonds, carbon credits, and sustainability-linked loans, has become increasingly accessible for companies meeting sustainability criteria. These financing models provide lower interest rates or grant-like funds to companies that actively reduce emissions, enhance waste management, or adopt fair labor practices (OECD, 2020). For mining companies, compliance with ESG standards can result in substantial capital cost savings, lower debt financing costs, and eligibility for grants and carbon offset programs, all of which strengthen financial flexibility.
​
For instance, as more investors prioritize ESG-compliant companies, mining firms can attract increased investment, resulting in enhanced market valuations and lower financing costs (GRESB, 2022). An example of ESG-aligned innovation is the use of advanced, sustainable technologies such as molten salt reactors (MSRs) for cleaner energy in mining processes. Incorporating MSRs or electrified mining machinery can help companies cut operational emissions while simultaneously reducing long-term energy costs. This aligns with studies suggesting that companies prioritizing ESG principles can anticipate a 15-20% increase in profitability by 2040 through efficiency gains, reduced environmental liabilities, and stronger investor trust (Haggerty et al., 2022).
​
The Economic and Social Benefits of ESG Adoption
Beyond financing advantages, adopting ESG practices can enhance a mining company's brand image, employee satisfaction, and regulatory standing. Sustainable initiatives, like utilizing nuclear or renewable energy sources, directly address emissions and improve the overall health and safety of both employees and local communities by reducing reliance on polluting fossil fuels. Companies investing in these technologies benefit from stabilized energy costs, shielding them from fossil fuel price volatility while establishing long-term, reliable energy supplies (BlackRock, 2020).
​
Socially responsible practices also translate to reputational benefits, as a strong ESG profile can make mining firms more attractive to investors, consumers, and potential employees. Companies committed to reducing their carbon footprint and improving working conditions often gain a competitive edge in recruitment, retention, and productivity, as employees increasingly prioritize values-driven organizations. Likewise, ESG-compliant companies face lower regulatory risks, as they demonstrate proactive compliance with environmental standards, reducing potential liabilities associated with fines or operational halts.
​
Positioning Mining Companies as Clean Energy Leaders
The mining sector's shift toward ESG compliance aligns with broader industry goals of clean energy leadership. By adopting innovative solutions like SMRs and other renewable technologies, mining companies can position themselves at the forefront of the global energy transition. This proactive role not only improves their operational sustainability but also helps the sector establish itself as a critical partner in reducing greenhouse gas emissions. The tangible financial, operational, and social advantages offered by ESG compliance are rapidly transforming sustainable practices into not only an environmental imperative but also a compelling business strategy.
​​
Conclusion: Mining's Role in the Global Energy Transition
The future of critical mineral mining lies in its ability to evolve into an industry that supports global decarbonization efforts while mitigating its own environmental impacts. Mining’s contributions to renewable energy technologies are indispensable, yet its sustainability challenges necessitate a transformation toward greener practices. By adopting low-carbon technologies, transitioning to renewable energy, and implementing ESG-compliant practices, the mining sector can significantly reduce its carbon footprint while benefiting from improved financial performance, regulatory compliance, and social acceptance. Nuclear energy, particularly through innovations like small modular reactors, offers promising pathways to decarbonize energy-intensive mining operations in remote locations, but its expansion requires rigorous safety and regulatory oversight. In prioritizing ESG standards, mining companies not only enhance their resilience and market appeal but also position themselves as integral contributors to the low-carbon future. Ultimately, achieving a sustainable balance between resource extraction and environmental stewardship is essential to meet the growing demand for critical minerals while supporting climate goals and fostering long-term industry resilience.
​​
References
-
Ali, S. H., Giurco, D., Arndt, N., Nickless, E., Brown, G., Demetriades, A., ... & Yakovleva, N. (2017). Mineral supply for sustainable development requires resource governance. Nature, 543(7645), 367-372.
-
BlackRock. (2020). Sustainable investing: Resilience amid uncertainty. Retrieved from BlackRock.com.
-
Copper Alliance. (2021). Copper’s role in achieving a low-carbon economy. International Copper Association. Retrieved from copperalliance.org.
-
EPA. (2023). Greenhouse gas emissions: Transportation sector emissions. U.S. Environmental Protection Agency. Retrieved from epa.gov.
-
FAO. (2022). The state of food and agriculture 2022. Food and Agriculture Organization of the United Nations. Retrieved from fao.org.
-
GRESB. (2022). GRESB Real Estate Assessment. GRESB. Retrieved from gresb.com.
-
Greenpeace. (2021). Mining and deforestation in the Amazon. Greenpeace. Retrieved from greenpeace.org.
-
Haggerty, J., Toombs, T., & Julian, D. (2022). ESG in mining and metals: Transitioning to a low-carbon world. Journal of Sustainable Mining, 21(4), 138-146.
-
IEA. (2021). The role of critical minerals in clean energy transitions. International Energy Agency. Retrieved from iea.org.
-
IEA. (2022). World energy outlook 2022. International Energy Agency. Retrieved from iea.org.
-
IEA. (2023). Global energy demand and emissions. International Energy Agency. Retrieved from iea.org.
-
IPCC. (2021). Sixth Assessment Report. Intergovernmental Panel on Climate Change. Retrieved from ipcc.ch.
-
McKinsey. (2022). The future of mining in a low-carbon world. McKinsey & Company. Retrieved from mckinsey.com.
-
Norgate, T. E., & Haque, N. (2010). Energy and greenhouse gas impacts of mining and mineral processing operations. Journal of Cleaner Production, 18(3), 266-274.
-
OECD. (2020). Green bonds: Mobilising the debt capital markets for a low-carbon transition. Organisation for Economic Co-operation and Development. Retrieved from oecd.org.
-
U.S. Geological Survey. (2022). Mineral commodity summaries. U.S. Geological Survey. Retrieved from usgs.gov.
-
UNEP. (2020). Sustainable infrastructure and the SDGs. United Nations Environment Programme. Retrieved from unep.org.
-
UNEP. (2022). Restoration and ecosystem resilience. United Nations Environment Programme. Retrieved from unep.org.
-
USGBC. (2021). LEED v4.1 Building Design and Construction. U.S. Green Building Council. Retrieved from usgbc.org.
-
Wood Mackenzie. (2021). Mining for a low-carbon future: Decarbonising the mining sector. Wood Mackenzie. Retrieved from woodmac.com.
-
World Bank. (2020). Minerals for climate action: The mineral intensity of the clean energy transition. World Bank Group. Retrieved from worldbank.org.
-
World Bank. (2021). The role of mining in the low-carbon economy. World Bank Group. Retrieved from worldbank.org.
-
World Nuclear Association. (2023). Small modular reactors (SMRs). World Nuclear Association. Retrieved from world-nuclear.org.
-
World Steel Association. (2021). Steel’s contribution to a low-carbon future. World Steel Association. Retrieved from worldsteel.org.
-
WWF. (2021). Gold mining and deforestation. World Wildlife Fund. Retrieved from wwf.org.